Biologicals are naturally occurring compounds or synthetically derived compounds that are structurally similar (and functionally identical) to their naturally occurring counterparts.
In general, biologicals are characterized by a non-toxic mode of action that may affect the growth and development of a pest, its ability to reproduce, or pest ecology. They also may have an impact on the growth and development of treated plants including their post harvest physiology.
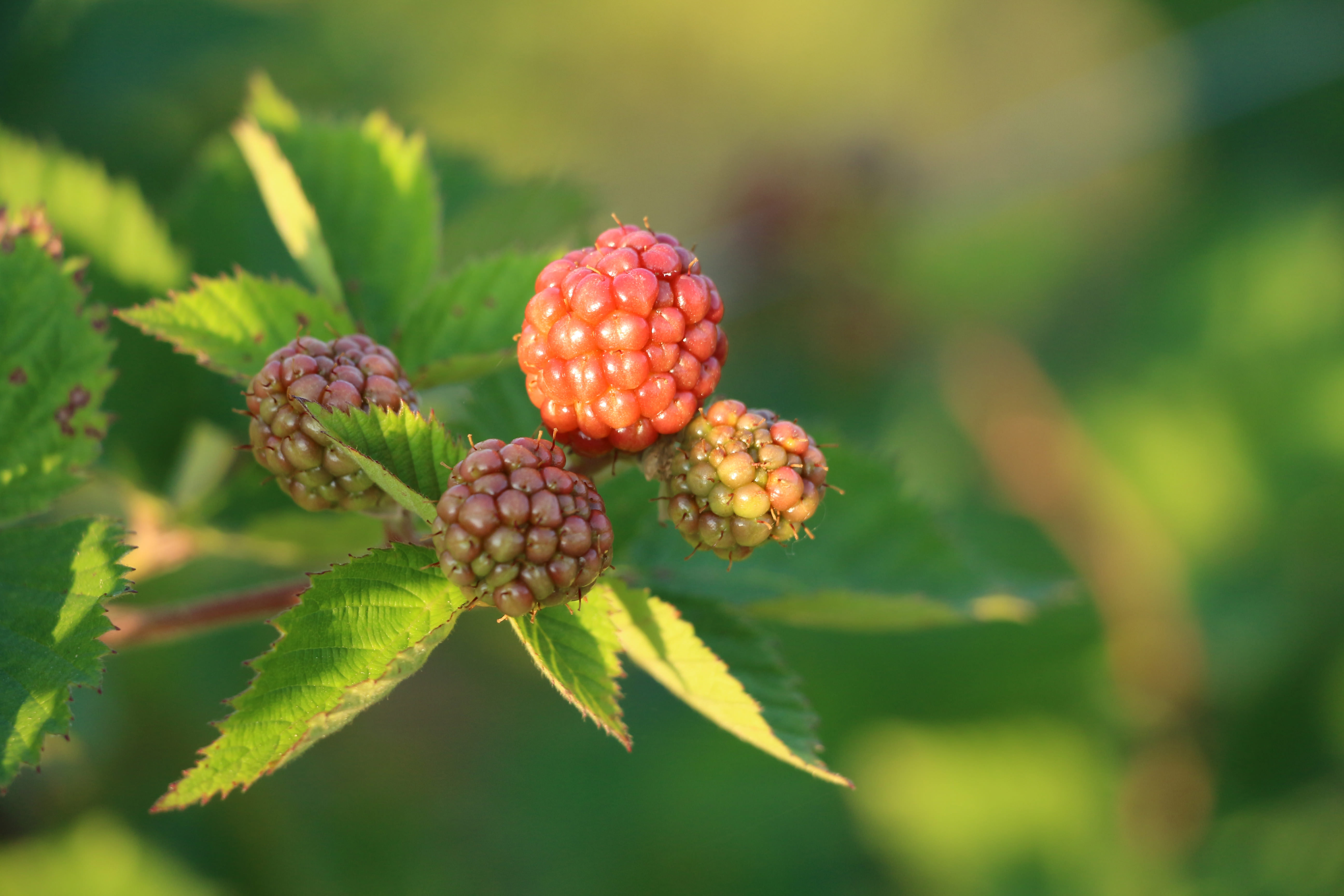
Biologicals are divided into several different subcategories of products, including:
Plant Growth Regulators
Plant Growth Regulators (PGRs) include a category of both natural and manufactured versions of natural substances that affect major physiological functions of plants. PGRs can promote, inhibit, or modify the physiological traits of a range of fruit, vegetable, ornamental and agronomic crops.
Once considered a rather esoteric practice, use of PGRs in mainstream agriculture has steadily increased as their benefits have become better understood by growers. From a crop production perspective, PGRs are used to maximize productivity and quality, improve consistency in production, and overcome genetic and abiotic limitations to plant productivity.
There are five major classes of natural plant hormones. Under each class there are a number of PGR products that play specific and very important roles in optimizing crop yield and quality.
- Gibberellins play a role in cell elongation, stem growth, bolting in long-day plants, induction of seed germination, enzyme production during germination, fruit setting and growth, and induction of maleness in dioecious flowers.
- Cytokinins are involved in growth promotion through cell division, fruit abscission, growth of lateral buds, leaf expansion, delay of leaf senescence, enhancement of stomatal opening, and chloroplast development.
- Abscisic acid can influence water relations via stomatal closure, inhibit shoot growth, inhibit alpha-amylase synthesis in seeds affecting dormancy, increase fruit coloring, and promote leaf and fruit abscission.
- Ethylene can stimulate lateral growth in stems, stimulate defense responses to injury, break dormancy, abscise leaves, flowers and fruit, induce flowering, and stimulate fruit ripening.
- Auxins can promote growth through cell enlargement, release apical dominance, and influence rooting, leaf senescence, flowering, fruit set and growth, fruit ripening, and leaf and fruit abscission.
In addition to the major five classes of plant hormones, there are other plant compounds with suggested PGR activity that are involved with cell division, maturation, cell enlargement, plant defenses and plant resistance. These compounds include a variety of secondary metabolites produced by plants and some plant-associated microbes. Examples include polyamines, brassinosteroids, jasmonates, salicylic acid and signal peptides.
There are a variety of concrete examples of how PGRs meet the needs of growers in the fresh produce market. These include:
Improved Fruit Quality
Gibberellins reduce russet on apples. Gibberellic acid improves firmness in cherries, and abscisic acid improves red color on red grapes. Ethylene is used to ripen fruit crops.
Improved Yield
Gibberellins increase fruit set on citrus and many other crops. Gibberellins increase leaf expansion on vegetable crops and cytokinins increase berry size on table grapes. And, ethylene modulation by aminoethoxyvinylglycine increases nut set on walnuts.
Overcoming Genetic Limitations
Gibberellic acid improves seed germination on dwarf rice varieties and increases berry size on seedless table grapes. Gibberellins and cytokinins can also increase fruit size in small apple varieties.
Reduce Labor Costs
Cytokinins and auxins induce thinning of flowers and fruits. Ethylene modulation by aminoethoxyvinylglycine allows growers to manage the timing of fruit maturity and, thus, the harvest process.
Expand Post Harvest Life
Ethylene modulation by aminoethoxyvinylglycine and ethylene receptor blockage by 1-methylcyclopropene can enhance fruit storageability. And, gibberellic acid extends the green life of bananas during transit and storage.
Insect Growth Regulators
Biobiological Insect Growth Regulators (IGRs) have a unique mode of action separate from most chemical insecticides. Generally speaking, these products prevent insects from reaching a reproductive stage, thereby reducing the expansion of pest populations. The direct impact of IGRs on target pests combined with the preservation of beneficial insects and pollinators aids growers in maximizing yield and product quality.
IGRs can be divided into two broad categories; i.e. those that disrupt the hormonal regulation of insect metamorphosis, and those that disrupt the synthesis of chitin, a principal component of insect exoskeletons . Agricultural applications currently focus on the first category of compounds, products which are also known as “hormone mimics.”
Azadirachtin is one of the most widely used botanical insect growth regulators. Because of its structural resemblance to the natural insect molting hormone ecdysone, azadirachtin interrupts molting, metamorphosis, and development of the female reproductive system. Immature insects exposed to azadirachtin (mainly by ingestion) may molt prematurely or die before they can complete a properly timed molt. Those insects that survive a treatment are likely to develop into a deformed adult incapable of feeding, dispersing, or reproducing.
Since beneficial insects, predators, and pollinators do not feed directly on the treated foliage, biological insect growth regulators are considered “soft” on beneficial insects such as honeybees, lady bugs, green lacewings, and the parasitic wasps.
Due to their unique mode of action, biochemical insect growth regulators have played an important role in integrated pest management systems and as an effective resistance management tool. A good example is the use of azadirachtin IGR in aphid population management programs for lettuce crops in the Salinas Valley, Calfornia. Due to restrictions placed on certain crop protection products, the lettuce industry has relied on the use of contact and systematic control measures – some with overlapping modes of action – which creates concern for pesticide resistance. Integrated use of azadirachtin provides control by impacting the larvae and nymphs of multiple aphid species, breaking the life cycle before they become reproducing adults.
Another azadirachtin success story is its use for pear psylla control on pears in the Pacific Northwest Region of the US, where growers integrate traditional control products, azadirachtin, and kaolin clay for a effective pest management with significantly reduced use of harmful chemical insecticides.
Organic Acids
Peracids are highly effective sanitizing agents used for control of pathogens and algae. Peracids, also called activated peroxygen, use hydrogen peroxide (H2O2) as their base component. Hydrogen peroxide is a well-known chemical compound that can be found in most people’s medicine cabinets for disinfecting cuts and bruises, among other uses.
Peracids combine hydrogen peroxide and organic acids, typically acetic acid, to form a new compound called peroxyacetic acid, abbreviated as PAA. This new compound is an activated form of hydrogen peroxide and produces a much more stable and powerful oxidizing compound to treat pathogens and algae in water.
The mode of action of peracids is by oxidation of cell membranes and penetration into cell structures of algae, bacteria and fungi. More specifically, peracids form free hydroxyl radicals (OH), which oxidize and disrupt thiol groups in proteins and enzymes.
Several features make peracids an excellent choice for agricultural and horticultural water treatment. First, peracid products that have been specifically formulated for horticulture offer a high degree of safety with regard to phytotoxicity. With formulated peracids, the grower can easily adjust the concentration of active ingredient in the treated water to account for increased biological loading. This is important since some organisms are easily susceptible even at low levels of peracids whereas others such as Pythium require higher active ingredient levels. Peracid products can be used for sanitation of greenhouse surfaces, shock applications for tanks and piping, continuous application at a low concentration, and also as a bactericidal or fungicidal application to plant foliage or roots. A further advantage is that when peracids degrade, the byproduct is oxygen, which is safe and beneficial .
Plant Extracts
Plants are, in effect, natural laboratories in which a great number of chemicals are biosynthesized. Many plants have developed natural, biochemical mechanisms to defend themselves from weed competition and animal, insect and fungal attacks. Some of these chemicals discourage feeding by insects and other herbivores. Others provide protection or even immunity from diseases caused by some pathogens. Still others help plants compete for resources by discouraging competition among different plant species. By studying the diverse chemistries of many different plant species, scientists have discovered many useful compounds that can be used as biologicals.
Plant extracts have long been used to control insects. Dating as far back as 400 B.C., children were deloused using a powder obtained from the dried flowers of the pyrethrum plant (Tanacetum cinerariifolium). The first botanical insecticide dates back to the 17th century, when it was shown that nicotine from tobacco leaves killed plum beetles. Today, there are a number of biocontrols plant extracts being marketed as insecticides. These products fall into several different classes:
Insect Growth Regulators
Plant extracts that prevent insects from reaching the reproductive stage.
Feeding Deterrents
A compound that, once ingested by the insect pest, causes it to stop feeding and eventually, to starve to death. Crop damage is inhibited and the insect eventually starves to death.
Repellents
Typically compounds which release odors that are unappealing or irritating to insects. Examples include garlic or pepper-based insecticides.
Confusants
Compounds that imitate food sources and are used as traps or decoys to draw damaging insects away from crops. Confusants can also be formulated as concentrated sprays designed to overwhelm insects with so many sources of stimuli that they cannot locate the crop.
Not only are plant extracts used directly as insecticides, but they are used also as a source for synthetic insecticides based on analogues developed in the laboratory. Scientists have modified molecules found in plants to be more toxic or more persistent. Common examples of this can be found in the pyrethroid and neonicitinoid families of insecticides, derived from molecules isolated from plants like pyrethrum (T. cinerariifolium) and tobacco.
Plant extracts can also be used as bioherbicides, and are based on several different modes of action:
Plant Allelopathy
A term used to describe the biochemical interaction that inhibits the growth of neighboring plants by another plant. Black walnut trees( Juglans nigra), for example, produce the allelochemical juglone, which is toxic to many other plants. Many recently discovered allelochemicals have potential for development as natural product herbicides.
Plant Growth Regulation
Some plant oils can act as effective contact herbicides through a variety of mechanisms such as disrupting cell membranes in plant tissue, inhibiting amino acid synthesis, or precluding production of enzymes necessary for photosynthesis.
Mechanical Control
Some plant extracts are powerful natural agents which act directly on weeds. D-limonene, for example, is an effective degreasing agent which strips the waxy cuticle from weed leaves, causing necrosis, dehydration and weed death.
Plant extracts are also used in commercial agriculture as fungicides and anti-microbial tools in food safety applications. They rely on the following modes of action:
Fungicidal Control
Some plant extracts act as contact fungicides. Some disrupt cell membrane integrity at different stages of fungal development, while others inactivate key enzymes and interfere with metabolic processes.
Induced Resistance
Crops treated with some plant extracts produce and accumulate elevated levels of specialized proteins and other compounds which inhibit the development of fungal and bacterial diseases. In effect, the crop’s immune system is triggered to defend against destructive diseases.
Using plant extracts as biocontrols offers growers many unique benefits. Generally, plant-based compounds degrade rapidly, reducing the risk of residues on food. Many of these products have very short preharvest intervals. Most products show wide windows of crop safety and resistance to these compounds is not developed as quickly as with synthetic pesticides due to multiple modes of action. Many plant extracts used as insecticides are fast-acting, quickly inhibiting insect feeding and additional crop damage. And, because they act on the insect’s gut and rapidly decompose in the environment, many plant extract insecticides are more selective to insect targets and safer to beneficial insects.
Pheromones
Pheromones are chemical signals that trigger a natural response in another member of the same species. Insects release pheromones to serve many functions. These include secretion of pheromones to to indicate the location of food sources, to warn others around about potential dangers, or locate a potential mate for reproduction. For example, female moths release a sex pheromone into the air to attract male moths. Male moths detect the pheromone “scent” and then follow it to locate and mate with the female. In agriculture, the problem is that mated female insect pests lays eggs and the emerging larvae damage crops.
Synthetic pheromones can be used in a number of ways to disrupt pest ecology and reduce crop damage. The first approach is to place small amounts of the female pheromone in lures to attract males into traps. By measuring trap counts, technicians can use that data to predict insect populations and decide when to implement the appropriate control methods.
Another, more common, use of synthetic pheromones is mating disruption. Growers saturate the environment with a sex pheromone so the male moths cannot easily locate females, disrupting their ability to mate. No insect mating means no fertilized eggs, and no larvae to damage crops.
Each year, over one million acres worldwide are treated with pheromones to control insect damage through mating disruption. Like many biologicals, synthetic pheromones are very specific, so they help maintain beneficial insects. They also provide additional benefits such as no re-entry intervals, no pre-harvest intervals, labor and harvest flexibility, worker safety, and environmental safety.
Minerals/Other
Minerals play a key role in a wide range of biological applications that can be divided into three categories:
- those that create barriers that keep pests from plant tissues and/or impact pathogen water supply
- those that deliver physical impacts such as smothering or abrasion
- those that act as an inert carrier for companion biologicals.
Kaolin clay is a good example of a biological that creates a physical barrier between insects and plant tissues. Kaolin acts as a repellent that coats the plant surface and makes it unsuitable for insect feeding and/or egg laying. Kaolin also breaks off in small particles that attach to insects, agitating and repelling them.
Potassium silicate is another example of this type of biological, which also serves as a dessicant to soft bodies insects and mites.
Diatomaceous earth (DE) is an example of a mineral biological that combats insect infestations through abrasion. DE contains fossilized microscopic plants, giving the compound a sharp surface that cuts through insects’ exoskeletons, a process that leads to dessication of the insect.
Mineral oils are used to smother insect pests in nesting stages.
Minerals are also used as inert carriers for companion biologicals. In these applications, minerals are included in formulations to deliver or enhance pest control agents, but the mineral itself is considered an inert ingredient. Examples of these mineral types include montmorillonite, attapulgite, kaolin, and talc, all of which have physical structures that allow biologicals to be carried on them, often in powder or granular formats.